
This illustration depicts a herringbone-like pattern in the atomic lattice of a quantum material created by researchers at SLAC and Stanford. An electronic tug-of-war between its layers has dramatically warped the lattice. Researchers are just staring to explore how this 'huge' distortion affects the material's properties.
UF, Stanford researchers make new type of quantum material with dramatic pattern
Created by an electronic tug-of-war between the material's atomic layers, this ‘beautiful’ herringbone-like pattern could give rise to unique features that scientists are just starting to explore.
Researchers have created a new type of quantum material whose atomic scaffolding, or lattice, has been dramatically warped into a herringbone pattern, described in a paper published today in Nature.
The resulting distortions are “huge” compared to those achieved in other materials, said Woo Jin Kim, a postdoctoral researcher at the Stanford Institute for Materials and Energy Sciences (SIMES) at the SLAC National Accelerator Laboratory who led the study.
Chunjing Jia, one of the paper’s authors, left Stanford last year to join the faculty as assistant professor in the Physics Department in the College of Liberal Arts and Sciences at the University of Florida.
“It has a lot of potenetial but it’s not clear at this stage what practical applications it may have,” said Jia, who was a leading theorist on the project.
“This is a very fundamental result, so it’s hard to make predictions about what may or may not come out of it, but the possibilities are exciting,” said SLAC/Stanford Professor and SIMES Director Harold Hwang.
“Based on theoretical modeling from members of our team, it looks like the new material has intriguing magnetic, orbital and charge order properties that we plan to investigate further,” he said. Those are some of the very properties that scientists think give quantum materials their surprising characteristics.
High-rises versus octahedrons
The herringbone-patterned material is the first demonstration of something called the Jahn-Teller (JT) effect in a layered material with a flat, planar lattice, like a high-rise building with evenly spaced floors.
The JT effect addresses the dilemma an electron faces when it approaches an ion – an atom that’s missing one or more electrons.
Just like a ball rolling along the ground will stop and settle in a low spot, the electron will seek out and occupy the vacancy in the atom’s electron orbitals that has the lowest energy state. But sometimes there are two vacancies with equally low energies. What then?
If the ion is in a molecule or embedded in a crystal, the JT effect distorts the surrounding atomic lattice in a way that leaves only one vacancy at the lowest energy state, solving the electron’s problem, Hwang said.
And when the whole crystal lattice consists of JT ions, in some cases the overall crystal structure warps, so the electron’s dilemma is cooperatively solved for all the ions.
That’s what happened in this study.

“The Jahn-Teller effect creates strong interactions between the electrons and between the electrons and the lattice,” Hwang said. “This is thought to play key roles in the physics of a number of quantum materials.”
The JT effect had already been demonstrated for single molecules and for 3D crystalline materials that consist of ions arranged in octahedral or tetrahedral structures. In fact, JT oxides based on manganese or copper exhibit colossal magnetoresistance and high-temperature superconductivity – leading scientists to wonder what would happen in materials based on other elements or having a different structure.
In this study, the SIMES researchers turned a material made of cobalt, calcium and oxygen (CaCoO2.5), which has a different stacking of octahedral and tetrahedral layers and is known as brownmillerite, into a layered material (CaCoO2) where the JT effect could take hold. They did it with a chemical trick developed at SIMES a few years ago to make the first nickel oxide superconductor.
Pulling out Jenga blocks
Kim synthesized a thin film of brownmillerite and chemically removed single layers of oxygen atoms from its lattice, much like players carefully remove blocks from a Jenga tower. The lattice collapsed and settled into a flat, planar configuration with alternating layers containing negatively charged cobalt ions – the JT ions – and positively charged calcium ions.
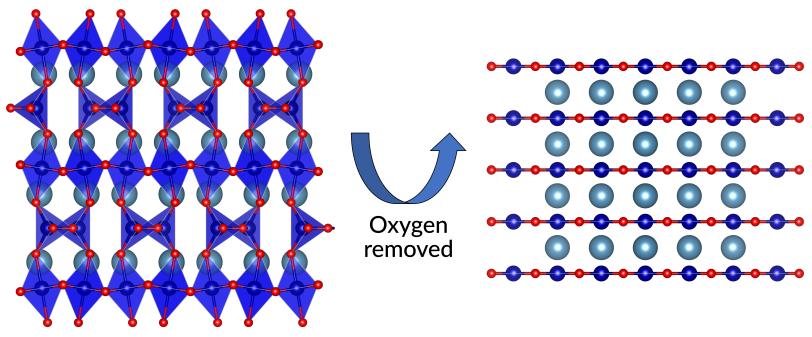
Each cobalt ion tried to pull calcium ions from the layers above and below it, Kim said.
“This tug-of-war between adjacent layers led to a beautiful pattern of distortions that reflects the best and most harmonious compromise between the forces at play,” he said. “And the resulting lattice distortions are huge compared to those in other materials – equal to 25% of the distance between ions in the lattice.”
Hwang said the research team will be exploring this remarkable new electronic configuration with X-ray tools available at SLAC and elsewhere. “We also wonder what will happen if we can dope this material – replacing some atoms with others to change the number of electrons that are free to move around,” he said. “There are many exciting possibilities.”
Researchers from Cornell University, the Pohang Accelerator Laboratory in South Korea and the Center for Nanoscale Materials Sciences, a DOE Office of Science user facility at Oak Ridge National Laboratory, contributed to this work. It received major funding from the DOE Office of Science and the Gordon and Betty Moore Foundation’s Emergent Phenomena in Quantum Systems Initiative.
Citation: Woo Jin Kim et al., Nature, 22 February 2023 (10.1038/s41586-022-05681-2)
This article was written by Glennda Chui at the SLAC Office of Communications at communications@slac.stanford.edu with contributions from the News and Publications Department in CLAS at UF.
SLAC is a vibrant multiprogram laboratory that explores how the universe works at the biggest, smallest and fastest scales and invents powerful tools used by scientists around the globe. With research spanning particle physics, astrophysics and cosmology, materials, chemistry, bio- and energy sciences and scientific computing, we help solve real-world problems and advance the interests of the nation.
SLAC is operated by Stanford University for the U.S. Department of Energy’s Office of Science. The Office of Science is the single largest supporter of basic research in the physical sciences in the United States and is working to address some of the most pressing challenges of our time.