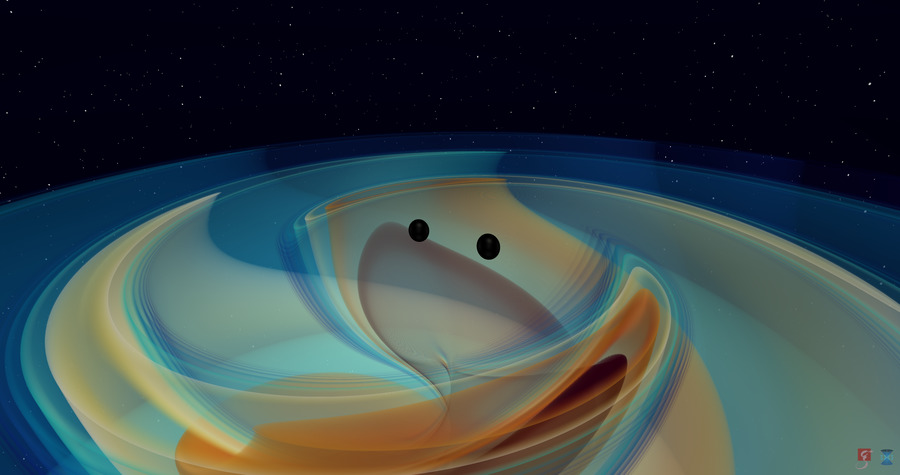
Simulated view of the impossible black hole and its companion before their collision, with their emitted gravitational waves. Credit: N. Fischer, H. Pfeiffer, A. Buonanno (Max Planck Institute for Gravitational Physics), Simulating eXtreme Spacetimes (SXS) Collaboration.
Research news: The black hole that should not exist, and other surprises from gravitational wave astrophysics
By Professor Imre Bartos
Going up to an old attic and turning on the light for the first time inevitably leads to surprises. Something similar is underway in gravitational wave astronomy. Since 2015, we have had a fundamentally new way to observe the universe, and the skeletons have already started falling out.
Gravitational waves are tiny ripples in space-time produced when a gravitating body accelerates. The strongest waves are created when two black holes collide. Their mutual pull makes these massive cosmic objects reach almost the speed of light before the crash creates a single, even more massive black hole.
The LIGO collaboration first discovered gravitational waves in 2015 using a search algorithm developed at UF. This first observation was already a surprise, having come only hours after the LIGO detector was turned on, hinting that black holes collide much more often than anticipated. It was the first of many surprises.
In 2017, LIGO found a different kind of collision: that of two ultra-compact, dead stars called neutron stars. This collision left a black hole in its wake and, in the process, also spewed out a large quantity of heavy elements, including gold weighing 5 times the mass of Earth. Using radioactive isotopes found in meteorites, UF-led research showed that a similar neutron star collision occurred near our solar system just before the system’s birth. This single collision is responsible for 0.3% of the gold and other heavy elements found today on Earth.
LIGO’s biggest surprise so far was a black hole collision observed in 2019 by a UF-led search algorithm. The collision included the heaviest recorded black hole. This black hole, whose diameter was as large as the state of Florida, was so massive that it should not have existed. We thought black holes were born when giant stars died. These stars create new elements through nuclear fusion. After consecutively heavier elements are produced through fusion, the star’s core can reach a critical density at which it collapses under its own weight. For the heaviest stars, no force of nature is strong enough to halt the fall, and eventually, matter reaches a density where its gravitational pull allows nothing, not even light, to escape, and a black hole is born. This process, though, does not work for the heaviest of stars. These stars burn so hot that they explode before they can collapse, leaving nothing behind but a bright explosion, a supernova. Based on the mass of the heaviest stars, this explosion limits the mass of a black hole that can be left behind to around 50 times the mass of our Sun. However, LIGO’s heaviest black hole was almost twice as massive as this limit, crying for a different explanation. Research led by UF and others indicate that this impossibly massive black hole could be the end result of a smaller black hole eating up multiple other small black holes, increasing the total mass above the single star limit.
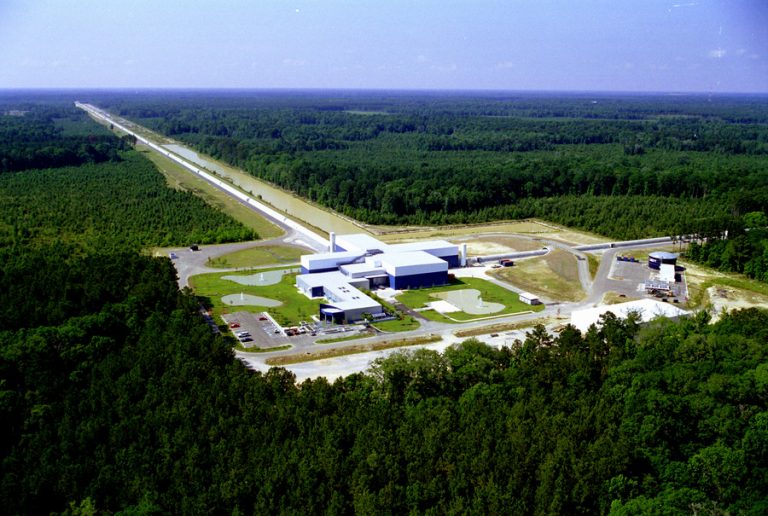
Now, UF and the gravitational-wave community have their sights on an even bigger hunting ground. The next generation of gravitational wave detectors will be an extraordinary leap. In the next decade, we will detect black hole collisions from virtually the entire observable universe. LISA, a space-based gravitational wave antenna under construction, will be able to detect some black hole collisions that occurred when the cosmic microwave background was created, so soon after the Big Bang that the universe was less than one ten-thousandth of its present age. For comparison, the universe was only 100 times younger than it is today when the first stars were born. This will open unprecedented windows into how the universe evolved over time, and even whether there could be exotic black holes, unrelated to stars, forged directly by the Big Bang itself.
Much remains to be done. UF researchers are currently working to trace the ancestry of LIGO’s numerous black holes, which could fill many otherwise inaccessible pieces of the cosmic puzzle. With the unparalleled and rapidly growing observational window we can access through gravitational wave observations, future surprises are all but guaranteed.
Imre Bartos is an associate professor at UF Physics and a recent recipient of a Sloan Research Fellowship.